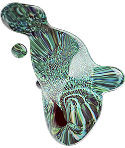
The science behind sensory intelligence
Life doesn’t begin with genes—it begins with responses.
In the early 1900s, Jagdish Chandra Bose, challenged the sharp boundary between living and nonliving matter, by finding that both metals and plants exhibit forms of excitability.
This suggested something radical but fundamental: that all matter can experience some form of sensation.
We believe that this capacity for sensation is the most overlooked aspect of the story of biological intelligence.
By understanding and harnessing the foundations of sensation, we can build machines that can make sense of the world, not merely simulate it.
All matter can sense: sensation begins with state change
The structured yet dynamic nature of matter allows it to have meaningful responses to external stimulation. Even the simplest molecules exhibit this capacity—suggesting sensation predates cognition.
Manfred Eigen won the Nobel Prize in 1967 for demonstrating that acoustic relaxation could resolve extremely fast chemical reactions.
His experiments revealed that molecular information is entangled in sound waves, and that reaction dynamics and energy dissipation are as fundamental to function as the molecule in question.
Later in his career, Eigen extended the idea that life is governed by physics and chemistry, by adding the concept of information to the equation.
Inspired by Eigen, Konrad Kaufmann applied this thinking to one of the fastest reactions in biology: acetylcholine clearance in the synapse.
Trying to reconcile these dynamics with thermodynamic constraints, Kaufmann proposed a radical idea: nerve impulses are not just electrical spikes—they are thermodynamic sound waves, whose form is dictated by the state diagram of the nerve membrane.
This thermodynamic perspective found experimental confirmation in the work of Matthias Schneider and Shamit Shrivastava (Apoha’s founder), who showed that Lucassen waves—nonlinear acoustic pulses—can travel along lipid interfaces near phase transitions with the same thermodynamic efficiency and properties as neuronal action potentials.
These waves are measurable and behave just like neurones in the human nervous system, even though they exist in inert materials, without any genes or proteins to guide them.
Sensation implies information: state change carries signals
Sensation is a measurable, high-dimensional imprint left on an excitable substrate by a physical perturbation. This imprint is structured, reproducible, and reveals the state-dependent response of matter.
This understanding began with physics: from Hans Bethe’s wartime work on shockwaves near phase transitions, to John von Neumann’s theoretical studies of detonation waves—each explored how matter carries and transforms energy in structured, propagating fronts.
Our founder, Shamit Shrivastava, later discovered that nonlinear waves propagating through matter near phase boundaries can act as both sensors and encoders of information.
Waves carry multi-parameter information on the chemicals that stimulated them. Lucassen waves in particular, respond both to physical state and local chemical conditions.
These waves are not passive—they are sensitive to both molecular identity and the medium’s energy landscape, allowing them to register and amplify microscopic differences into measurable macroscopic effects.
After decades of research into the possibility of sensory intelligence, in 2021, in partnership with Thermofisher Scientific, Apoha built a proof of concept that showed that waves can easily do highly sensitive anomaly detection in chemical steam.
This was able to measure differences that other detectors are blind to.
Simple systems can respond to state change signals and process information
In the Liquid Brain, both the substrate and the molecular input are perturbed as they interact. The resulting wave-driven imprint captures the molecular properties that are otherwise difficult to isolate or quantify.
Using principles of analogue computation in physical media., the Liquid Brain® can then convert these raw dynamics into stable, high-dimensional biophysical signatures.
We see sensory intelligence as a form of physical processing that turns stimuli into temporal structure, where information is not encoded into bits, but emerges from dynamic interactions between molecules.
This insight was central to the early ideas of John Hopfield, who showed that molecular proofreading, memory, and pattern recognition in biology rely on dynamic processes, not explicit instructions. Decoding the multiparameter information that is encoded in waves is therefore a computing problem.
Systems like reservoir computing formalised how nonlinear, high-dimensional physical systems—from water tanks to optical media—can process information just by being perturbed.
Biophysical classification is a hard problem at the heart of materials science: you can’t design new drugs or materials if you don’t know how they might behave.
In 2024, Apoha successfully used reservoir computing of its Liquid Brain® to decode the behaviour of different molecules, matching the total performance of 12 other leading biophysical classification assays.
We have since learned how to mine 1000x more useful information from a measurement (preprint out in May 2025).